
This year we are organizing a "2 in 1" event as our fourth quarter event. Combining both seminar and networking in one day to ease our fellow concrete lover for arranging their time.
The seminar is organized to nourish the participants with the knowledge related to latest innovations in concrete construction, materials and systems in line with the objectives of the Chapter. This invitation is extended to engineers, architects, consultants, academics, contractors, suppliers, builders, as well as to anyone with the interest in cement and concrete technology.
On the other hand, the networking session will be held after the seminar. Buffet dinner will be served and more importantly we get to mingle with fellow concrete enthusiast.
For more details, download the brochure
2018 ACI-MALAYSIA SEMINAR ON CONCRETE AND
CONCRETE STRUCTURES
&
NETWORKING DINNER
This year we are organizing a "2 in 1" event as our fourth quarter event. Combining both seminar and networking in one day to ease our fellow concrete lover for arranging their time.
The seminar is organized to nourish the participants with the knowledge related to latest innovations in concrete construction, materials and systems in line with the objectives of the Chapter. This invitation is extended to engineers, architects, consultants, academics, contractors, suppliers, builders, as well as to anyone with the interest in cement and concrete technology.
On the other hand, the networking session will be held after the seminar. Buffet dinner will be served and more importantly we get to mingle with fellow concrete enthusiast.
For more details, download the brochure
2018 ACI-MALAYSIA SEMINAR ON CONCRETE AND
CONCRETE STRUCTURES
&
NETWORKING DINNER
This year we are organizing a "2 in 1" event as our fourth quarter event. Combining both seminar and networking in one day to ease our fellow concrete lover for arranging their time.
The seminar is organized to nourish the participants with the knowledge related to latest innovations in concrete construction, materials and systems in line with the objectives of the Chapter. This invitation is extended to engineers, architects, consultants, academics, contractors, suppliers, builders, as well as to anyone with the interest in cement and concrete technology.
On the other hand, the networking session will be held after the seminar. Buffet dinner will be served and more importantly we get to mingle with fellow concrete enthusiast.
For more details, download the brochure
2018 ACI-MALAYSIA SEMINAR ON CONCRETE AND
CONCRETE STRUCTURES
&
NETWORKING DINNER
This year we are organizing a "2 in 1" event as our fourth quarter event. Combining both seminar and networking in one day to ease our fellow concrete lover for arranging their time.
The seminar is organized to nourish the participants with the knowledge related to latest innovations in concrete construction, materials and systems in line with the objectives of the Chapter. This invitation is extended to engineers, architects, consultants, academics, contractors, suppliers, builders, as well as to anyone with the interest in cement and concrete technology.
On the other hand, the networking session will be held after the seminar. Buffet dinner will be served and more importantly we get to mingle with fellow concrete enthusiast.
For more details, download the brochure
2018 ACI-MALAYSIA SEMINAR ON CONCRETE AND
CONCRETE STRUCTURES
&
NETWORKING DINNER
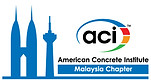
American Concrete Institute
Malaysia Chapter
My Items
I'm a title. Click here to edit me.
Wet Crack Injections in Basement Slabs Explained
Written By: Mr. James Lim Date: 21 Apr 2022 Wet crack repair is becoming an integral part of concrete design to manage cracks that are prone to water seepage, especially in the lowest basement slab where water pressure is high. In today’s construction design, the base slab or the raft foundation of a building is often designed with waterproofing admixture with the intention to waterproof the base slab against ingress of ground water. The use of waterproofing admixture is seemingly a trend now as structural engineers prefer admixture over the traditional membrane system. In order for it to work, the concrete has to be perfectly placed, vibrated properly so that a honeycomb and crack free concrete is produced at the end of day. However, the reality often gives a different outlook of this concept of admixture waterproofing system because it fails to address the natural occurrence of cracks in concrete structures. Whether it is based on pore blocking or formation of crystals that are only visible under the microscope, the truth is that the journey down this path is not so smooth sailing after all when cracks start to develop. All the so called self-heal mechanism of the admixture in the concrete will quickly evolve into a discussion of crack mapping and repair methodology not to mention who will be bearing the cost of the cracks repair. When cracks start to develop, coupled with high water table of our ground condition, it does not take long for us to realise that the basement slab will soon become wet. Water ingress from the ground is almost inevitable in this scenario and there is only one way out from here. The objective has become clear that the project manager of the job site is now looking at the best way to seal those cracks so that the leaking cracks will not leak again. This might sound like a daunting task for a project manager as now he has to look for money to perform this task. Main contractors will probably agree that injection grouting is the way to solve these problems relating to water ingress at the basement slab. Structural engineers prefer the cracks to be sealed with epoxy resin as it gives strength and good bond but majority of the epoxy resin in the market caters for dry crack condition. Epoxy resin is good for dry cracks normally found in intermediate floor slab. In the case of a base slab, epoxy resin will not be effective to seal against ingress of water from the ground. So a polyurethane (PU) based injection resin is preferred. Having said that, the majority of the polyurethane resin in our market consists mostly of the foaming type of PU resin (1K) and this normally come in a single pack water reacting resin. Water acts as the catalyst to start the reaction when the PU resin is injected into the wet cracks thus creating a foam that is filled with air bubbles. The foaming PU resin is normally formulated with a foaming factor between 20 to 40x. The higher the foaming factor, the lighter the foam as there are more air bubbles within the foam structure. As it is a foam, it does not come with strength and the foam can easily break or shrink over time. The German injection methodology treats this type of injection with the PU foam as temporary seal. So if you were to get a recurring leak after the injection, the PU foam has either shrunk and failed to resist the water pressure thus allowing water to escape through the cracks again. If someone says PU does not work, that basically means he has bad experienced with foaming PU resin. What’s next if you have lost faith in PU injection? It is perhaps time to look at dual component PU technology. The formulation of a two-component (2K) PU resin allow the mixed resin to achieve a compressive strength of up to 80MPa which was previously not possible with 1K PU. A 2K PU resin will set after mixing the two separate components together without the presence of water. This is an important criteria of a good resin as not all parts of the wet cracks are wet and the PU resin will still set inside the crack if it finds a dry patch. The PU resin in the dry section will set just like an epoxy resin giving it good bond and compressive strength. Unlike foaming PU, the resin will not set in locations without the presence of water because water is required to catalyze the PU resin to start the foam reaction. There are really no real advantages of using foaming PU resin to seal any wet cracks once you understand the chemistry of a foaming resin. The only reason why foaming resin is widely used is due to cost factor as the resin is much cheaper compared to a 2K resin. But does it really save money for the project manager? The project manager shall look at the overall cost of the injection repair when it comes to wet crack repair rather than ask for a per point injection rate from the specialist contractor. Allowing your applicator to quote you based on a per point basis type of injection contract is like signing on a blank cheque and allowing your applicator to fill in the amount. Can you imagine the damages this concept can do to your project’s bottom line? In conclusion, concrete admixture alone is insufficient to prevent water ingress as a result of crack formation. Perhaps then, it is time to switch to a two-component injection resin system for a permanent fix. - Thank you -
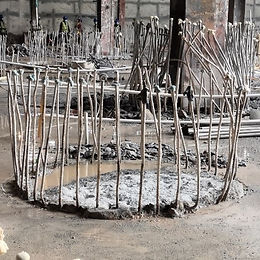
Pile Head Treatment: A 2-in-1 Grouting Proposition
Writer: Smith Yong Date: 28/12/2021 Pile foundation is one of the important structural elements in a building construction. The primary function of pile foundation is to transfer the loads from the structure above to the more compact, solid and stiffer soil which have higher bearing capacity to withstand the structural load efficiently. Typically, reinforced concrete piles are widely use in Malaysia. Pile foundations system which commonly used in Malaysia market are reinforced concrete square piles, spun piles, bored piles, micropiles and etc. Deep basement construction is getting common in Malaysia especially at the city centres due to rapid development of the city landscape coupled with scarcity of land for new development. When the structure extends far below grade, the challenges of waterproofing work increase exponentially as the hydrostatic pressure increases. Without efficient basement waterproofing system, issues relating to water seepages could lead to serious structural damages in the long run due to corrosion of the steel reinforcement. Concrete element in building is reinforced with steel reinforcement or wire mesh. Failure of waterproofing system may lead to ingress of water to the concrete structures. Exposure to air and water leading to corrosion of the steel reinforcement and wire mesh which eventually will result in structural damage over time. Therefore, special care and attention are essential when selecting the appropriate waterproofing solution for basement structure. Pile head waterproofing integrity is critical in deep basement construction and careful attention must be given to ensure no leakages occur. In comparison to basement slab, pile head waterproofing work is more difficult to treat as it involves lots of detailing work especially around the congested steel reinforcement area. Water can seep through the basement structures along the reinforcement steel if it is not treated properly. Selection of reprofiling material for pile head treatment is very important as the material must be able to provide not only waterproofing properties, but also able to withstand the heavy loads from the whole building. Therefore, the pile head waterproofing treatment system shall be integrated with the basement slab to form a watertight system. At the construction site, pile head trimming is a process is to prepare the pile head to pile cut-off level and expose the reinforcement for incorporation into the pile cap. After the trimming process, the pile head will have an undulating surface as shown in the photo below. Thus, pile head reprofiling is required prior to the pile cap casting work. It is very common to see “non-shrink” cementitious grout being used as the material to reprofile the pile head. “Non-shrink” cementitious grout is chosen because it can be easily source in the market and have high compressive strength. It is considered as an economical way to grout the pile head. However, is “non-shrink” cementitious grout the right material for the work methods employed in the above application? Photo 1: Uneven finishing of pile head after trimming process “Non-shrink” cementitious grout is a single component material which contains cement, graded fillers and other additives. The cement acts as the binder and upon adding water, it forms a high strength cementitious material. Is “Non-shrink” cementitious grout really does not shrink? The market term “Non-shrink” grout is commonly referred to because they contain special additives which cause volume expansion either during the plastic stage and/or the hardening stage to counter the shrinkage during curing process. For precision grouting and formwork repair applications, water lost during the curing process is well-controlled as the material is applied into a confined space and the exposure area of the grout surface is small. This application allows the grout to have a proper curing process without significant change of volume and thus minimize the formation of shrinkage cracks while maintaining a good bond with the substrate. However, if “Non-shrink” cementitious grout is applied on expose hot weather and low humidity environment, it is difficult to control the loss of moisture during curing and that leads to rapid hardening of the grout. Improper curing may result in permanent damaging effects to the cement based “Non-shrink” grout. This gives rise to shrinkage cracks, lower compressive strength, poor adhesion and etc. Furthermore, it is very challenging to ensure proper execution and protection work on site to avoid rapid drying issue. As with all cement based material, it does not have the “waterproof” property which this essential for pile head treatment in order to prevent the ingress of ground water from the piles. If there were any defects found on the pile head surface, rectification work is mandatory prior to basement slab casting work. What’s next if “Non-shrink” cementitious grout is not suitable for pile head reprofiling work? It is perhaps time to look at epoxy grout. Epoxy grout is three components, high performance grout that derived from an epoxy based binder and a filler material. Epoxy grout use epoxy instead of cement as binder, thus making it to be waterproofed since epoxy is an impermeable material. Its zero water penetration properties provide a full waterproofing barrier to the pile head. In addition, epoxy grouts offer rapid curing, high early compressive strength and high ultimate compressive strength development. In terms compressive strength, epoxy grouts can achieve approximately 60MPa in one day and 90MPa in 7 days whereas “non-shrink” cementitious grout can only achieve approximately 30MPa of compressive strength in one day and 65MPa in 28 days. At the job site, basement construction is always under the critical path of the construction schedule. Any delay along the critical path may affects the overall completion time of the project. With epoxy grout, the foundation contractor is able to shorten his hand over timeline to the main contractor for subsequent rebar work due to the shortened time to achieve the required compressive strength. “Non-shrink” cementitious grout takes longer time than epoxy grout to achieve its expected strength as per material data published by product manufacturer. “Non-shrink” cementitious grout takes 28 days to achieve full cure whereas epoxy grout only takes 7 days to achieve full cure condition. If the “Non-shrink” cementitious grout is not cured properly, it can lead to the formation of shrinkage cracks and thermal cracks. As a result, the durability and strength of the grout as well as the bonding to the piles will be compromised. In contrast, epoxy grouts do not have shrinkage issue. This is one of the major benefits of epoxy grout over “Non-shrink” cementitious grout. When shrinkage and thermal cracks start to develop, coupled with high hydrostatic pressure from the ground water, water ingress from the ground will keep the basement structure wet over time. Injection grouting is the only way to solve this problem. It is impossible to hack off the existing pile head and redo the waterproofing work after the basement structure is completed. The only reason why “Non-shrink” cementitious grout is widely used is due to the cost factor. The basement slab waterproofing system is also incomplete without considering the pile head treatment in relation to water ingress issues. Perhaps we shall look at the overall cost instead of the upfront cost. When it comes to basement slab leaking repair work, the amount of time spend for investigation work and the remedial cost by the specialist contractor could be quite significant. Pile head treatment is the final and most crucial application step for a successful watertight basement structure which is often overlooked. Every single pile has to be treated properly to prevent any leakage through the top of the pile. In conclusion, the differences between epoxy grout and “non-shrink” cementitious grout are summarised as table below: Table 1: General Comparison of epoxy resin grout and “Non-shrink” cementitious grout. Photo 2: Pile head treated with epoxy grout - Thank you -
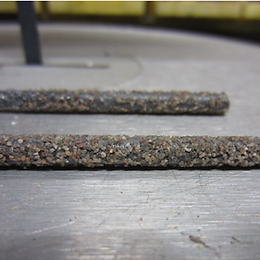
An Overview of CFRP Reinforcement in Concrete
Date: 23/10/2021 Writer: Dr. Shobana Sivanendran Derived from excerpts of the PhD thesis of Dr. Shobana Sivanendran, University of Cambridge, 2017 Traditionally, concrete structures are internally reinforced with steel bars. However, there are many problems that result from the corrosion of this steel reinforcement. The corrosion products of the steel reinforcement occupy a greater volume than the original steel causing bursting forces to build up within the concrete cover. This leads to cracking and spalling of the concrete, which could significantly weaken the structure. In prestressed concrete, corrosion could lead to sudden snapping of the pre-tensioned steel reinforcement, which has in some cases led to catastrophic damage of large structures. In 1985, a post-tensioned single span concrete bridge in Ynys-y-Gwas, Wales, collapsed suddenly with no signs of weakening prior to failure. The failure was attributed to severe localised corrosion of the prestressed tendons. To this day, there has been extensive research conducted to find ways of mitigating this problem of corroding steel reinforcement. The addition of silica fume to concrete mixes significantly decreases the permeability of the concrete to water. This makes it more difficult for external moisture to penetrate into the concrete and instigate the corrosion process in the steel reinforcement. However, it does not prevent moisture from penetrating through cracks in the concrete. To solve this, research has also been conducted to find ways to initiate a self-healing process for cracked concrete that could prevent environmental moisture from reaching the reinforcement. However this idea is still in its infancy and has yet to produce crack healing at the required rate and robustness for field applications. The other main alternative to preventing corrosion in reinforced or prestressed concrete is to replace the steel entirely with a non-corrosive material. Following from the successful applications of fibre reinforced polymer (FRP) materials in the aeronautical and maritime industries, the idea for the use of such materials as construction materials has been explored. The three fibres commonly considered were glass (GFRP), carbon (CFRP) and aramid (AFRP). The main challenge in using FRP rods as a replacement for steel lies in their inherently different material properties. FRPs fail in a brittle manner. This is unlike steel, which yields significantly prior to ultimate failure i.e. steel fails in a ductile manner. Therefore if a concrete structure were to be under-reinforced with FRP rods, there could be limited warning signs if the rods were close to their ultimate load capacity. Particularly for prestressed FRP rods, any failures could occur suddenly. Additionally, FRPs have a much higher strain capacity than steel, approximately 10 times higher, and are also significantly more expensive. Therefore the most economical method of utilising them would be as prestressed reinforcement. These considerations mean that a different design approach to that of steel reinforced concrete is required when using FRPs. While CFRP rods with a high strength and stiffness offer an attractive alternative to steel as prestressed reinforcement because of their non-corrosive nature, other modes of deterioration of these rods when exposed to high moisture environments cannot be ruled out. Any deterioration over the long-term should be accounted for during the design stage to ensure safe structures for the duration of their design life. The CFRP tendons used in pre-stressed applications are cylindrical in shape with diameters ranging from 3mm to 10mm. They are made of thousands of continuous unidirectional carbon fibres of diameter approximately 5-6µm set in an epoxy matrix. The tendons are most commonly manufactured using the pultrusion method, where the carbon fibre strands are pulled through a resin bath and then through a heated die thus forming a continuous carbon-epoxy tendon. They are then surface treated to encourage better bond with the concrete. Spiral wound fibres, indentations and sand coatings may be added during the manufacturing process to improve the CFRP-concrete bond. Bundles of rods may be combined to form strands, also used in prestressed concrete members. The tendons derive most of their strength from the fibres while the matrix functions primarily as a binder of the fibres and a distributor of the imposed loads. Because of the unidirectional orientation of the fibres, the resulting polymer is anisotropic and possesses different material properties in the longitudinal and transverse directions. Material properties in the direction parallel to the fibres are fibre dominated and material properties in the transverse orientation to the fibres are matrix dominated. Thus when considering the durability of CFRP tendons, it is important to take note of these directional effects in relation to the directions of the applied loads. Currently in Malaysia, there are some applications of CFRP sheets for the strengthening of concrete structures, however the use of CFRP as internal prestressed reinforcement in concrete is still yet to be applied. Potentially with further research, the benefits of such applications for structures that are more prone to corrosion, such as sea bridges, could be better understood to pave the way to the use of CFRP as internal prestressed reinforcement here. Images: Image 1: Photograph of sand-coated CFRP tendons (Source: Dr. Shobana Sivanendran) Image 2: 500x optical microscope photograph of transverse cross section of CFRP rod showing the carbon fibres and epoxy matrix (Source: Dr. Shobana Sivanendran)
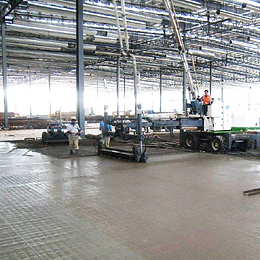
How Big A Concrete Floor Slab Can Be Casted Without Cracks?
This article is to discuss what is the optimum size a concrete floor slab can be casted in a single panel without any cracks and defects especially in the industrial floor sector which demand for high quality floor. Even though the technology behind the construction of concrete slab has improved over the years, besides expecting a floor to be built flat and level, one of the major challenges facing the construction industry is how to built a ‘crack-free slab. Concrete being a vagarious material tends to crack due to various reasons but mainly caused by drying shrinkage, thermal movement and restraints. Therefore, concrete floor cracking may be caused by a single factor but also combination of two or more factors. As such designers and builders can only endeavor to mitigate, minimize, or control cracks but almost impossible to guarantee constructing a large concrete slab with no cracks. 1.0 Why big slabs are preferred? If slabs are casted in smaller panels and without restraints, cracks can easily be avoided but to construct a large size panel, for example 1,000m2 in a single pour successfully without any form of cracks and defects will be a challenge. Large slabs are preferred as it speed up the job, contain less construction joints and contraction joints which are potential weakness on the slab if not properly constructed as shown in Figure 1. The other advantage of minimum joints is to reduce curling of slab at edges as shown in Figure 2. Such curling defects will disrupt smooth operation of lift-trucks and spalling at joints resulting in continuously costly repair. 2.0 Construction of concrete floor slab (slab-on-grade) Concrete has high compressive strength but low tensile strength and tensile strength of the concrete is only about 10% of the compressive strength, therefore concrete will cracks when the tensile stress within exceeds the tensile strength of the concrete. Hence, one way to mitigate cracks is to lower the tensile stress in concrete and to increase the tensile strength of the concrete. When cracks occurred, blame goes to poor workmanship, poor quality of concrete supplied or improper design. In actual facts, any cracks resulted could be man-made or caused by factors to be discussed as follows. Common factors which may contribute to cracking in floor slab. 2.1 Ground settlement Subgrade is the original ground and it is best to carry out a soil investigation to study the soil bearing capacity to support the intended design load. Any filling materials selected for filling low shall be suitable for easy filling and well compaction. A well-compacted sub-base will facilitate loaded vehicles to roam the construction site freely without rutting the surface and also a simple proof rolling method to ensure the subgrade and sub-base are well compacted to prevent settlement cracks. 2.2 Contraction joints As thicker slab is stiffer and will tend to produce less cracks compared to a thinner one unless the later is specially designed. Likewise, a thinner slab will also tend to curl more than a thicker slab of similar mix design and working condition. Therefore, to avoid random cracking, contraction joints are created on weaken planes on the slab surface at locations where high stresses accumulate and prone to cracks. In fact, contraction joints are simply pre-determine man-made straight line cracks created to release stresses on the floor surface to induce cracks when the slab commence to shrink, being straight line man-made cracks, nobody will complain. If contraction joints are not provided to disipate the stresses, random cracks will be unavoidable.The contraction joints are carry out using mechanical diamond saw machines the next day or the same day if a green blade is available. The depth of cut is normally one-fourth of the slab thickness and the saw-cutting is carried out immediately when the concrete gained sufficient hardnesss, normally between 8 to 12 hr after the floor is finished. To mitigate random cracks, contraction joint spacings of maximum 36 times the slab thickness, up to a maximum of 5.5meter have produced acceptable results(ACI 302.1R-04). If a slab is 150mm thick, the contraction joints need to be spaced at every 5.4meter. Contradictly, wider contraction joints spacing of up to 16m x 16m have been successfully carried out reinforced with 20kg/m3 of steel fibres without any form of cracks. 2.3 Type of reinforcement and positioning To select welded mesh or steel bars as reinforcement for crack control is one task but placing and positioning the reinforcement location right is the challenge. The purpose of reinforcement is to provide additional strength where needed and if cracks happened, it supposed to keep the cracks tight and preventing it from opening and becoming wider. If the location of reinforcement is wrongly placed, for example, placed lower than mid section of slab, it will defeat it’s purpose and may not performed. It is best to position about 30mm from the surface for crack control. To overcome such difficult to position the reinforcement right, steel fibres or synthetic fibres are used as a replacement for better crack control. Generally, fibres will provide better crack control as they are 3-dimensionally distributed within the concrete slab to absorb any tensile stresses which occur at early stage when the young concrete has yet to achieve the tensile strength to resist cracks. When early micro-cracks developed, the fibre strand will intersect and blocked the micro-cracks from developing into macro-cracks as the concrete shrinks. 2.4 Concrete mix design Concrete mix design is of utmost important not only to produce high strength concrete but to achieve good workability, cohesiveness, durability and minimum shrinkage to mitigate cracks. The main cause of cracks resulted from volumetric changes of concrete as the water evaporates gradually resulting in shrinkages. As a rule of thumb, every 6m of concrete slab, can shrink as much as 3mm, as such it is advisable to use lowest permissible water/cement ratio for the concrete mix design without affecting the workability as water being the single greatest factor affecting shrinkage. Research confirmed that 1% increase in water will increase shrinkage by about 2%. When the concrete is still in the plastic phase, the concrete surface dries and shrinks so rapidly that tensile strain exceed the strain capacity of the young concrete which will results in plastic shrinkage cracks. Designing a concrete mix right with minimum shrinkage of less than 3% will certainly reduce drying shrinkage cracks and with high early strength will avoid early thermal cracks. Another important consideration is the type of cement used as Ordinary Portland Cement (OPC) will achieve initial set faster with early strength compare to supplementary cementitious materials like pulverised fly ash added. Therefore, one way to design concrete using minimum water without affecting the workability of the concrete by selecting and packing graded fine aggregate, coarse aggregate with selective superplasticizer in the mix. For construction of floor slabs, the recommended cement content using OPC shall be below 400kg/m3 to avoid curling of slab. 2.5 Poor detailing. Design error and poor detailing will exposed the slab to greater stresses than it can handled, resulting in cracking. For example, cracks propagated from corners of columns (as shown in Fig. 3) was caused by diagonal re-bars being omitted (shown in Figure 4). Such re-entrance corner cracks happen in all floor openings and columns where stress concentration are high. 2.6 Slab size and aspect ratio of width/length Slabs are generally casted in narrow strip, wide bays or large pour. The recommended aspect ratio of width/length is preferably 1:1 and not to exceed 1:1.5 as mentioned in “Guide for Concrete Floor and Slab Construction”, ACI 302.1R80. Any floor panels with excessive width-to-length ratios as mentioned will tend to crack to release stresses at the mid-panel surface or at locations with excessive stresses. However, this recommended width-to-length ratio could only be extended to 1:2 or more subjected to better understanding of crack control, adding special admixture to reduce stress in concrete like shrinkage compensating admixture. Conservatively, recommendation of each panel size to be casted as follows. For slab on grade: External slab, each panel 4.5m x 4.5m with contraction joints. Internal slab, each panel 6.0m x 6.0m with contraction joints. For slab on piles: each cast 20m x 20m For elevated slabs: each cast 20m x 20m There is no guarantee that no cracking will be observed based on the abovesaid recommendation as concrete cracks can be considered normal, even though in practice, there were claims that slabs which were 2 to 3 times larger than above- mentioned have been done successfully. 2.7 Jointings - Isolation joints, contraction joints, etc For slab-on grade, casting of slabs can be carried out in large pour within a day but contraction joints (e.g. 6m x 6m interval) need to be performed to induce cracks within the slab to avoid random cracking. When the slab gradually shrinks and contracts, the cutted joints will slowly open up. As for construction joints for slab-on-grade, dowel bars need to be installed to cater for load transfer. As for suspended slabs, all construction joints need the reinforcement bars to be continuous. As for slab on piles, movements joints or expansion joints need to be designed at probably every 30 to 50m to facilitate expansion and contraction due to panel shrinkage and temperature change. 2.8 Restraints of slab For a slab on grade floor built on a well-compacted ground with reasonable flatness and levelness will allow the slab to slide freely with the sub-base with no surface restraints. As for suspended slabs, restraints will mainly be from the columns, beams, reinforced walls and openings. For slab-on-grade there are several forms of restraint that prevent the concrete from shrinking freely in the form of internal or external forces as follows. i. Internal restraint Normally for thicker slab (normally about 1 m thick) where thermal cracks can occur due to temperature difference between the surface zone and the internal core of the concrete. The surface of an element will cool faster (contraction) than the hot core (expansion), thus resulting in early thermal cracks and delayed entriquette formation if the temperature difference between the core and the surface exceeds 20◦C. ii. Surface restraint The resistance to allow the slab to slide freely onto the existing sub-base can be achieved by better flatness control on the sub-base surface. To further reduce the coefficient of friction between the slab and the sub-base, placing a slip membrane using polyethylene sheets with thickness of 0.25 mm is highly recommended besides acting as a moisture barrier. iii. End restraint Cracks often occurs when floor slab is not permitted to move freely especially when the floor reinforcement is tied to walls, beams and columns. For slab-on-grade, it is best to isolate the floor slab from such fixed structures to release any stresses. Likewise, all four ends of a floor panel should be debonded wherever possible from the ground beams or walls. iv. Edge restraint When casting strip pour (long and narrow slab) try to avoid casting the entire infill slab (panel between two completed adjacent slab) at one go if it is too long (> 30 meters), alternatively contraction joints if permitted should be allowed to control any transverse cracks. 2.9 Curing Curing is the process of controlling the rate and extent of moisture loss from concrete by trapping the water needed for the chemical reaction during cement hydration. Curing allows the concrete to achieve its potential strength and durability. Late curing or no curing is often cited as a major factor that caused surface defects like craze cracks and loss of concrete strength as much as 30%. As such, early and proper curing is essential to achieve the concrete strength needed and to improve abrasion resistance. Finally, to reduce drying shrinkage, proper curing should be carried out for at least 7 days as 80% of ultimate shrinkage is caused by inadequate or no curing. 2.10 Early thermal cracking During the hydration process when the cement reacts with water, the chemical reaction produces heat. In hot countries, compounded with the direct heat from strong sunlight onto the slab surface, it will caused the temperature of the slab to rise, resulting in thermal volume change which expand the slab. Subsequently, during the night when the concrete cools, it contracts and since the tensile strength of the concrete being relatively low may lead to cracking. 2.11 Shrinkage control Ordinary Portland Cement concrete shrinks as it dries, this shrinkage if restrained is conducive to cracking. Therefore, to eliminate drying shrinkage cracks, a shrinkage compensating cement is added to make the concrete expand vey slightly (about 0.0045%). This shrinkage compensating cement is designed to provide dimensional stability during the concrete curing period when the young concrete with low tensile strength is susceptible to crack when the tensile stress exceeds it. This shrinkage compensating cement will expand very slightly when added into concrete which compensate the drying shrinkage as it begins to dry. As the concrete sets, it bonds to the steel reinforcement and while the concrete is curing and gaining strength, the expansive reactions provide a slight controlled elongation to the concrete. The bond to the reinforcement steel causes it to be stretched slightly and places the steel in tension. Whilst the reinforcement steel is in tension, the concrete is thus put in slight compression which is favourable.With shrinkage compensating concrete, not only it will minimize cracks but the joint spacing can also be double. 3.0 Conclusion There is no arbitrary limit on the size of the slab to be poured in a single day if reasonable crack width is allowed and acceptable but to build a large slab without any form of cracking and defects is the biggest challenge. The maximum size of pour shall be determined by practical considerations such as the followings: 1. Can the concrete supplier provide continuous supply of concrete without any interruption to avoid defects like cold-joints. 2. Does the contractor have sufficient well-trained manpower with good construction practices and technical know-how to execute such large slab. 3. Are there sufficient, “state of art” machinery and equipment to fulfil the objective and meet the specified floor requirement. In conclusion, to construct a large floor slab without or minimum cracking, a composite concrete system is recommended by adding shrinkage compensating compound, lower water/cement ratio, heavier reinforcement and follow the best construction practices.
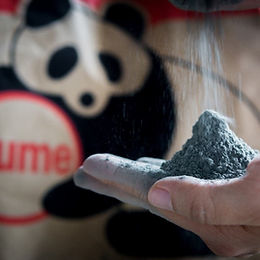
How To Choose The Right Cement?
C ement is a common material in construction industry. It is so common that sometimes people do not border to study it. When you ask someone “What is cement?", probably he/ she will just answer “A grey powder to bind materials together”. The Malaysian Standard MSEN 197-1 defines cement as a hydraulic binder, i.e. a finely ground inorganic material which, when mixed with water, forms a paste which sets and hardens by means of hydration reactions and processes and which, after hardening, retains its strength and stability even under water. It is a versatile substance that is primarily used to bind aggregates together in mortar and concrete. When it is mixed with fine aggregates and water, it produces mortar. When it is mixed with fine and coarse aggregates, water and admixtures, it forms concrete. Portland cement was developed in England by bricklayer Joseph Aspdin in 1824. It gets its name from its resemblance upon hardening to the famous Portland limestone obtained from quarries on the Isle of Portland. Portland cement is the most important cement in terms of the quantity produced. The main “raw material” to produce Portland cement is Portland cement clinker. Portland cement clinker is produced by heating a mixture of lime, iron, silica and alumina in a rotary kiln at temperature up to 1450oC. When the materials sinters, it partially fuses into clinker balls. The clinker balls are then cooled and ground to a fine powder together with a small amount of gypsum and the resulting product is Portland cement. Cement Standards and Types of Cement There is no universal international standard for cement because every country has its own standards. For example, the cement standard used in Malaysia is MSEN 197-1 (Malaysian Standard for Cement - Composition, specifications and conformity criteria for common cements) which is adopted from the European Standard. There are 27 types of common cement specified in MS EN 197-1 and they are grouped into 5 main cement types as below: CEM I Portland cement CEM II Portland-composite cement CEM III Blastfurnace cement CEM IV Pozzolanic cement CEM V Composite cement Although MSEN197-1 is the main cement standard used in Malaysia, the American Standard ASTM C150 (Standard Specification for Portland Cement) is also sometimes being specified in some projects. Both MSEN 197-1 and ASTM C150 classified cements into 5 main types namely Type I to V. However, the way these two standards classifying the type of cement is very different. MSEN classification is based on composition while ASTM classification is based on potential phase composition and application. Below is a summary of the types of cement classified in both standards for easy reference. In addition to the type of cement, it is important to know the strength class of the cement you are using as well. There are 3 standard strength classes specified in MSEN 197-1 i.e. 32.5, 42.5 and 52.5. On top of that, 3 classes of early strength (2 days or 7 days) are included for each class of standard strength, a class with ordinary early strength, indicated by N, a class with high early strength, indicated by R and a class with low early strength, indicated by L. Class L is only applicable for CEM III cements. These are the distinct low early strength blast furnace cements. Table below shows the strength requirement for each strength class specified in MSEN 197-1. Cement is sold both in bulk and bagged. The bagged cements sold in Malaysian market are generally classified into three types, namely the Ordinary Portland Cement, Portland Composite Cement and Masonry Cement. The Portland cements are general purpose cement which can be used for structural works and have to comply to MSEN 197-1. Masonry Cement which is used for plastering work has to comply to MSEN 413-1 (Malaysian Standard for Masonry Cement - Composition, specifications and conformity criteria) which is specifically for Masonry Cement. Masonry Cement is good for plastering work but cannot be use for structural work due to its low strength. In order to differentiate the Masonry Cement from the Portland cements, it is commonly associate with red bag in the packing. Factors to Consider Different types of cement have different characteristics. The most important variables are the rate of hardening, the strength gain pattern, the heat of hydration, the resistance of the hardened cement to sulfate chloride attack and other durability aspects. Construction documents often specify a cement type based on the required performance of the concrete or the placement conditions. Factors to be considered when selecting the right cement are : a) Type of construction b) Speed of construction c) Compressive strength requirement d) Strength development e) Specific requirements such as chloride & sulfate environment, permeability tests, control of ASR, etc. Although the process for cement manufacturing is relatively similar across the world, the standards requirement can be vary. There are different types of cement and sometimes it can be difficult to determine the suitable cement for your project. When ordering cement for construction projects, do not extrapolate performance data for one cement as representing all cements. Work with your cement supplier to verify that the cement is suitable and meeting the requirements for the project and application. Thank you

Concrete Technology 101
C oncrete is perhaps the most important construction material other than steel for the construction fraternity. It is easy to develop a specific type of concrete at the university laboratory but the story is vastly different when the same concrete is placed at the construction site in a bigger volume. So it is important to understand the different components of concrete and how the different components interact within the concrete mix. Traditionally, concrete is made of cement (Ordinary Portland Cement – OPC) + Water + Sand (Fine Aggregate) + granite stones (coarse Aggregate). For ready mix concrete, concrete admixtures such as retarder and superplasticiser are normally added to allow for transportation and maintain concrete specification. Cement (OPC) is the binder or the glue that bonds the fine and coarse aggregates together when both are mixed together. Water acts as the activator of the cement and help the transportation of the cement in a paste form around the aggregates so that a bond is created between the different ingredients inside the mix. The sand (fine aggregates) fills the voids in between stones (coarse aggregates). When the cement paste is set and given enough time to cure, concrete in its hardened state is formed. For the understanding of concrete enthusiasts, a basic design of concrete mixes is explained below: First of all, concrete that uses Ordinary Portland Cement (OPC) as the binder, can achieve a design strength of up to 60 N/mm². Beyond this, special additives will have to be added. Generally, concrete strength starts with grade 15 N/mm². For every increase of 5 N/mm², it is considered one grade higher. Now certain “Rule of Thumb” needs to be known and they are briefly explain as follow: - · 10kg/cement 1N/mm² · Water to cement ratio (WC ratio) not more than 0.5 (unless otherwise prescribed). · Approximately 20% of cement will not hydrate in the mix and will act as fillers. · Sand to stone ratio (SA ratio): 0.4 to 0.45 · 1m³ of concrete is about 2400 kg/m³ Assuming a Grade 30N/mm² concrete is required the calculation for the mix per m³ is as follows: - · Cement 30 x 10 = 300kg/m³ · Added 20% to compensate for the cement not hydrated. · Therefore cement content is 360 kg. · Water ( wc ratio) is 0.5 x 300kg = 150 kg/m³. · The cement and water weight are added to become 510 kg . · Since 1m3 of concrete weighs around 2400 kg, the weight of the stone + sand is 1,890 kg. · Now how much sand and how much stone to be used? · So we use the SA ratio of 0.45 . We multiply 0.45 x 1890 kg (sand + stone) · Therefore sand will be 850.5 kg and stone will be 1039.5 kg. · In summary the theoretically grade 30 concrete mix will be Cement : 360 kg Water : 180 kg Sand : 850.5 kg Stone: 1039.5 kg Now we have to look at the basic admixtures which are retarders and superplasticiser. Without them, commercial supply would be impossible. Concrete without retarders start to set after 30 minutes once it is mixed and around 1½ to 2 hours for final set. This will make transportation and workability impossible unless the batching plant is located within the construction site. In most cases they are not. So retarders will have to be added into the concrete mix to prolong the initial set to approximately 2 hours and final set approximately 5 to 6 hours. The retarder works by lining the cement particles thereby not allowing the water to activate the cement. After the retarder time has become worn off, it will then allow the water to be in contact with cement and start the activation process. Keeping in mind that overdosing may cause issue with non-setting. The superplasticiser is mainly acting as a water reducer where by you can maintain workability without increasing water content and compromising on the strength of the concrete. How this works is that the superplasticiser lines the cement particles with a negative charge thereby repelling particles from each other, thus avoiding clumps and releasing trap water in the cement clumps. Water can be reduced by 25% approximately. In today’s technology, concrete is produced in automated or semi-automated batching plants. The pictures below give an idea on the production flow. Once the concrete is place, a poker vibrator is used to vibrate to compact the concrete and to release the air trapped inside the concrete. In order to work on the concrete, the concrete must not be too wet or too dry. In order to check the concrete before placing to ascertain the design slump which indicates it has been produce according to design specification. A slump check is done. To ensure that concrete has met its design, a compressive test is carried out 28 day after production. This article aims to provide a basic understanding of the concrete mixes. For more specific mix design, a more in depth understanding of concrete and others influencing factors are as important to achieve the intended outcomes. You may consult the professionals.

The Relevance Of Tensile Strength & Elongation Of Waterproofing Membranes
These properties are often headlined as important features of waterproofing membranes: a) tensile strength b) elongation at break How relevant are these properties for waterproofing performance? In order to answer these questions, it is necessary to first look at these definitions: Elastic Deformation and Elastic Limit An elastic material deforms when a force is applied to it. So long as the deformation stays within the elastic limit, the material will revert to its original shape when the force is removed. Many waterproofing materials which are described as elastic do not show perfect elastic behaviour i.e the recovery is not 100 % after the force is removed. Plastic Deformation A plastic material also deforms when a force is applied to it. However, when the force is removed the material will not recover its original shape. Many plastic materials used for waterproofing exhibit a small recovery but not enough to justify calling them elastic. Elastic materials which are stretched beyond the elastic limit but before the breaking point will deform plastically i.e. with little or no recovery. Young’s Modulus of Elasticity, E Young's modulus measures the stiffness of elastic materials or the force needed to deform an object along an axis when the force is applied along that axis; it is measured as the ratio of stress to strain (change in length over the original length). Comparing two materials with differing E values, the material with lower modulus requires less force to elongate it by a defined amount. In other words, and this is relevant for assessing waterproofing membranes, the material with higher modulus is stressed more for the same elongation compared to a lower modulus material. a) How important is the tensile strength? For fully-adhered membranes, consider the stresses imposed on it when the supporting substrate moves due to, for example, settlement or temperature changes. Under these circumstances, the membrane’s tensile strength is irrelevant because its function is not to restrain the movement (it cannot, in any case), but to deform and accommodate the movement without suffering functional damage. Comparing two materials installed on the same structure: (i) The membrane with a lower tensile strength and lower E modulus may well fulfill this function and retain its waterproofing integrity so long as the movement is within its deformation limit, (ii) The other material, on the other hand, with a higher tensile strength and higher modulus (stiffer) may fracture because the elevated stress needed to deform it exceeds its tensile strength. Or, if the built-up tensile stresses overcome its adhesion strength, it will delaminate, allowing the lateral migration of water between the membrane and the substrate in case of leaks thus compromising its waterproofing performance. In general, tensile strength is important during handling and installation of pre-formed membranes and for loose-laid or mechanically-fixed membranes. b) How important is the elongation value? The ability of waterproofing membranes to stretch and elongate under stress is undoubtedly important in applications where the substrate is subjected to movement such as on roof slabs. However, the elongation value, taken in isolation, is often misleading. Superficially, a material with 800 % ultimate elongation looks far superior to another with 300 % elongation. But conside r these points: Very high elongation values are usually achieved by materials with plastic deformation behaviour or elastic materials which have been stretched beyond their elastic limits. This raises two concerns- (i) Over the expected s ervice life of the waterproofing membrane, stress cycles in the
support structure are repeated countless times. Therefore, plastic elongation with
little or no recovery does not measure the membrane’s performance durability; (ii) Elongation is achieved at the expense of the membrane thinning out. For materials
subjected to very high elongation, this thinning effect would have stretched it way
past its useful thickness well before breakage occurs. In view of the above, waterproofing membranes are often reinforced with fabrics; these are built-in for preformed sheets and added during installation for liquid-applied membranes. They serve to add elasticity (recovery property) and/or restrict the elongation within useful limits. Therefore, for a waterproofing membrane applied on structures subject to movement stresses, the elongation value on its own is not sufficient for assessing its fitness for purpose. The important performance property to look for is its dynamic crack-bridging or crack-cycling capability, tested to recognised standards eg EN 1062-7. This test measures the membrane’s ability to elongate and recover over repeated cycles, simulating conditions you would expect the membrane to undergo during its service life. (Reference must be made to the manufacturer’s technical data sheet for instructions on use of particular products.) - Thank You -
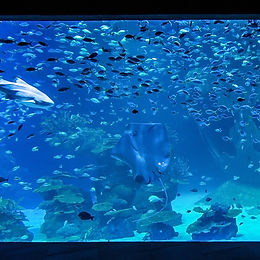
Built For The Oceans
T he oceans cover 71% of the world’s surface and contain 97.2% of the Earth’s water. With the increase of human population, effects of pollution on the oceans have also drastically increased, putting ecosystems and fish stock in jeopardy. According to the United Nations, many marine species worldwide are affected by marine debris, and as much as 80% of that litter is plastic. One of the best platforms to educate future generations about the effects of pollution on our planet are public oceanariums. They offer a “true world experience” in a controlled environment, which can be used for creating awareness, learning and inspiring change of habits for a cleaner environment. Bringing the ocean into a building is not a simple task. A huge amount of technology and husbandry know-how is required to bring an ocean ecosystem into an oceanarium tank. Construction methods to build these specialized concrete tanks require specific needs in order not to destabilize the exhibit’s water parameters while combating any possible structural deterioration from seawater. Fig. 1a: Oceanarium 3 million litres per hour non-metallic recirculation system Fig 1b: Oceanarium automated filtration system Seawater contains about 3.5% soluble salts (chlorides and sulfates) by weight and has a pH of around 7.5 to 8.4. It is a catalyst for corrosion, and like any exposed concrete structure to seawater in nature, direct exposure may deteriorate concrete through the effects of various chemical and physical processes. For example; • Sulfate attack • Leaching of Lime (Calcium hydroxide) • Alkali-aggregate expansion • Salt crystallization from wetting and drying • Corrosion of embedded reinforcing Controlling or stopping the deterioration through these processes is best done by reducing concrete permeability. Low permeability not only prevents tank leakage but, it also helps to keep seawater out of the concrete, thus slowing leaching and protecting the reinforcement steel from corrosion. Newly laid bare concrete, in whatever form, leaches when submersed in seawater. Leaching is very dangerous and lethal for an aquarium inhabitant causing spikes in pH and leaching of chemicals into the exhibit’s water column. The trick to building a successful oceanarium tank is a good waterproofing tank system and most importantly, to get it right the first time, as not to jeopardize the fishes when introduced into the tank. An oceanarium concrete tank structure can be grouped into 3 exposure zones; submerged, splash and atmospheric. The submerged zone is continuously covered by seawater, the splash zone is subject to wetting and drying and the atmospheric zone is exposed to seawater spray. Concrete in the submerged zone is not as vulnerable to corrosion compared to the 2 other zones. Oxidation works slower when submersed, but the flip side is that the leaching of lime can be fatal to fishes. Moreover, if metallic corrosion does happen underwater, the effects can be detrimental to some species, affecting the nervous and navigation system of most elasmobranchs (sharks and rays). Damage of concrete due to deterioration is like cancer; it slowly eats into your reinforced structure and spreads from there, increasing porosity and permeability to the concrete. There are multiple ways and systems available in the market that reduces permeability in the concrete but nothing beats a good lining system. Choosing a high strength, low permeability concrete mix with a minimum reinforcement cover of 50mm and a good non-toxic lining systems (eg. pure polyurea etc.) goes a long way towards creating a maintenance free and long service life concrete in a marine environment while keeping the fishes safe from contamination. Although a good design mix is a good start in ensuring a long concrete life, one must not forget that design and construction practices will affect strength, permeability and durability. We have found that a good lining system and low permeable base concrete base makes the worries go away. The reliability that every inch of concrete is placed properly in good construction practices can be a worrisome task and not practical for large tanks and areas. In addition, probable issues of external groundwater damages in structures below the water table are inherently difficult to identify. Lining systems like pure polyurea have revolutionized the construction and waterproofing of oceanarium tanks because it reduces the risk of permeability problems and the quick cure time allows work for other trades to continue efficiently. Fig 2a: Poorly placed new reinforced concrete tank that required rectification. Fig 2b: New reinforced concrete with lesser than 50mm cover Like with any protection membrane installation, a good base concrete is extremely important, as it (the lining) would be considered as the first line of defense and concrete cover as the second, before seawater reaches the reinforcing. In addition to this, high-risk areas for corrosion in seawater such as construction joints can be mitigated. It is found that with systems like these, localized rebar corrosion from construction joints and cracks is reduced drastically. Fig. 3a: Typical concrete tank surface preparation Fig. 3b: Pure polyurea waterproof lining system application In order to have a composite system to fully work together, the chemical bonding of lining and concrete plays a very important role. Proper moisture mapping, surface preparation and application are key in the laying of a 3mm pure polyurea lining system. Other key procedures such as viewing panel silicone bonding test between polyurea lining and the acrylic prior to installation, helps ensure all these material components are fully integrated and watertight. Fig 4: Silicone chemical bonding test to acrylic viewing system and waterproofing lining system, where the best primer is selected Fig. 5: Completed tank with aquascaping and fish (inhabitant) introduction In conclusion, bringing the ocean and encapsulating it into a tank is truly a unique multi faceted task and its poses a unique set of problems to overcome. When building an oceanarium, it is important that designers are fully educated in the nature of seawater and the importance of the details in specifying marine structure corrosion mitigation systems. Having the right specialist from the start is also the utmost importance, where proper structural mix and lining system are selected with experienced on-site quality control managed, so that inhabitants of the oceanarium is safe and the nature of the oceans can be truly appreciated. - Thank you -
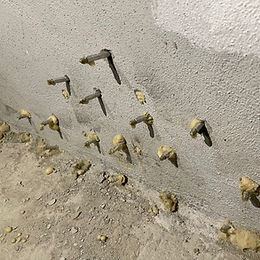
Crack Injection: For Show or For Real?
#crackinjection #pugrouting #puinjection #injectionpacker #injectionpump #seepage #wetcrack #epoxyinjection #resingrout W hen we observe a crack on concrete slab or beam, we can either patch it with something to close it or we can seal it with a high strength resin which provides compressive strength and bond strength. For this, engineers prefer epoxy resin because it has strength and good bonding properties. Most epoxy resin available in the market caters for dry cracks condition as epoxy resin will not set when it is mixed with water. For wet cracks, either underwater epoxy resin or high strength polyurethane resin shall be selected for crack injection. In this respect, 2 components polyurethane resin is ideal as it will react with the water to form a stiff material with a bonding strength of 1.5 N/mm2. It is important to know that structural polyurethane resin can achieve a compressive strength of 80 MPa and this is equivalent to the strength of an epoxy resin. Upon contact with water, polyurethane resin forms a rigid structure that does not allow air and water to pass through the cracks. When the cracks are sealed and air tight, corrosion of the steel reinforcement can be prevented. At the job site, it is not difficult to spot a group of injection nipples or injection packers sticking out along the line of crack to illustrate the repair job is completed by visual inspection. Now the question to ask is whether the resin has penetrated into the cracks as intended by the structural engineers who specified the method of repair. Surprising enough, nobody seems to be interested in asking whether the cracks are sealed properly. The sight of seeing rows of injection nipples or packers basically confirmed that the job was done as intended. So it is basically good showmanship because we don’t normally questioned whether the cracks have been properly sealed with injection resin or not. If you were to conduct a random test to check the resin penetration by core sampling test, you will notice most of the cracks are not filled with resin due to poor injection methodology. As engineers are not trained to recognized whether the specialist applicator has done a good job, the outcomes are often left for imagination. This is because the showmanship of crafting the rows of injection packers were suffice to confirm the job was done to reasonable standard. No further tests or quality control are put in place to ensure the resin actually penetrate the cracks. So real injection or great showmanship? The easiest way of finding out whether the injection is good is by looking at the way how the holes were drilled. The drilled hole is the only passage for the resin to travel into the crack. As simple as it may seems, drilled holes that are not drilled deep enough to intercept the cracks are likely to result in a road block situation. Road block means injecting resin into a solid wall of concrete because the holes were too shallow. To intercept the crack effectively, a drilling angle of 45 degrees are recommended versus drilling perpendicular to the concrete surface because the cracks are often not straight when you take a cross section view of the cracks. For method that uses surface packer or injection nipple where only epoxy adhesive is used to bond the nipple to the concrete, the injection resin could not travel far into the cracks because surface packer or nipple are not designed to handle high injection pressure. Low injection pressure limits the penetration depth of the resin and it is likely to result in incomplete filling of the cracks or even no fill at all if the packers/nipples were not aligned with the crack. Repair specialist prefers this method because it is quick and easy to do since drilling is not required. But it is often more of a showmanship to show the structural engineer that injection has been done to seal the cracks. What can we observe from the site picture taken above? 1. Do you need that many holes for an injection job? What surprises the most is that the contractor was even allowed to drill so many holes in a small area. 2. Inconsistent drilling angle. The bottom row is drilled at an angle and the upper rows were kind of perpendicular to the wall. Potential honeycomb location to inject perhaps. 3. Resin rebound back at the location of the packer and this indicates a road block situation. Therefore, injecting into solid concrete. 4. Injection packers were left on site. Perhaps to show proof of injection by showing the number of packers installed. With a perfect hole that connects the cracks to a high pressure piston injection pump, the chances are you will not require to drill as many holes as seen in picture above. The one with the most number of packers are the likely outcome of an amateur contractor with good showmanship. Unless the structural engineer requires to fill and bond the cracks properly, the injection shall be done with diagonal drilled holes staggered at both sides of the crack and then inject it with a high strength resin. With good drilled holes achieved, the required injection pressure should not be more than 1000 psi at the start and it will quickly drop to 300 psi when the resin starts to flow into the cracks. The concept of injection consists of an understanding of 3 elements below: 1. Connecting the cracks/zone of injection with a drill hole. Mostly like a longer drill bit to hit the spot. 2. Injection pressure. The key is to achieve consistent injection pressure during the process of injection. 3. Injection resin mix ratio and setting time. An ultra-fast setting resin requires a plural pump set up and built-in flushing capability. As simple as it might seems, real injection requires careful planning of packer position, resin selection and choice of injection pump. Asking the right questions in the process of resin selection and injection methodology will allow the engineers to get a better outcome when it comes to crack injection. -Thank you-
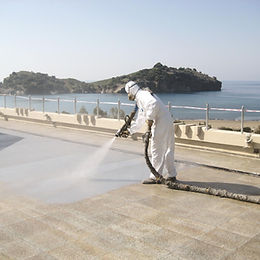
Roof Waterproofing Trends and Polyurea Solutions
C onstructing a roof, be it a concrete or metal roof can be an easy task for a typical roofing contractor. Concrete roof slabs are usually built with a waterproofing system that is designed with conventional system such as crystallization admixture, exposed EPDM membrane, liquid applied membrane such as acrylics and polyurethane coatings. Other than concrete roof, metal decking roof is also popular in factory construction where the metal sheet naturally provides the waterproofing properties it needed for a factory set up. Metal sheets overlap each other and fasteners provided the anchoring requirement to hold the metal deck in place. Concrete are prone to cracks due to shrinkage and cracks could compromise the waterproofing membrane at the flat roof. Similarly, metal deck and metal screws corrode over time and this could lead to issue relating to water seepage. This can be a pressing issue when the roof starts to leak and a solution is required. Started in the early 80’s, standard roof coating systems use to treat concrete roofs from further leaks include cementitious coating, torched on Bituminous Membrane, rolled on acrylics coatings and polyurethane coatings. Conventional coatings like these while they offer certain benefits, would not be considered a long-term solution today. Most of them could not be exposed to UV directly as UV’s will degrade the system causing premature failure of the coating or membrane. Epoxies and cementitious coatings are very rigid and are not designed to handle cracks due to poor elongation properties. Epoxies are likely to chalk and polyurethanes will degrade when exposed to the tropical climate with high UV and thermal expansion. In addition, the majority of the waterproofing membranes require a layer of protection screed to be laid over it. When this conventional system fails and the roof starts to leak, an exposed waterproofing with high abrasion resistance and stable under the UV is ideal for a re-waterproofing purpose as you do not have to remove the existing screed and waterproofed membrane and just lay the new membrane over it. Introducing Polyurea. Polyurea is a spray on elastomeric system which can be applied on both concrete and metal roof to resolve problem associated with water seepage. Polyurea can be applied to a thickness of 2-3mm, which will then give you an elongation property above 300% and a tensile strength of >15Mpa. Sprayed as a single coat system, it eliminates joints and overlapping within the coating itself and hence reducing the chances of leak from happening. As a roof coating repair system, it can be sprayed directly on existing surface after sufficient surface preparation is done, thus reducing the need to hack and remove existing screed which could be costly and time consuming. Furthermore, the shut down time of the affected areas can be reduced to a minimal due to the quick set nature of the polyurea resin, typically in less than 2 minutes after sprayed. Nevertheless, polyurea does have its limitations. The application of polyurea requires the operator to be skilled and well trained as the operation requires multiple set up of spray machine, air dryer and air compressor. The cost of maintenance of the spray machine and accessories can be high as the machine are supplied by niche vendors in the market. Depsite all the advantages it brings, the polyurea system also comes at a higher cost compared to conventional waterproofing system. The cost factor of this system often discourages wide spread use of polyurea for customer who understand its cost benefit analysis. The growing trend of green roofs being designed and built in the country also mean that conventional waterproofing with joints/seams will not work as root penetration attack on the coating itself can be an issue. In this case, polyurea are ideal as many product manufacturers have test their polyurea for anti-root penetration. Among other typical uses of polyurea coating includes portable drinking water tank lining systems which required local authority drinking water certificates, theme park coating where coatings are subject to constant immersion of chlorine and UV exposure as well as foot traffic.
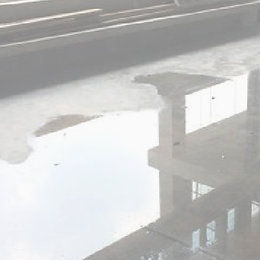
Is Water Ponding on Carpark Floors Normal?
Nowadays water ponding has become a common construction dispute as any water appearing stagnant on the floor surface us generally not acceptable by owners and/ or architects. Hence, is water ponding normal or should it be rectified? T wo issues are commonly encountered when rectification is made to water ponding. Firstly, if the affected area is rectified, there is a tendency that a new puddle of water my be created adjacent to the rectified area. Secondly, it is very difficult to carry out thin topping repairs on low areas successfully. Any mason can repair a floor but what is the assurance that the repaired area will not delaminate or fail months later? Before understanding the causes and finding a solution, everyone should know the difference between 'flat' and 'level'. Flatness relates to the bumpiness of the floor surface and levelness relates to the tilt or slope of the floor. To describe a floor profile clearly, flat and level are two inseparable entities with different types of floor profile as shown in Fig 1. Water ponding is common on concrete floor surfaces, especially at today's large carpark areas. This problem is unavoidable as most finished floor levels are specified to meet a 'perfect level', and even on a floor that is constructed to be super-flat and super-level, the water will still stagnate on the floor surface as the water has nowhere to fall. Therefore, the only way to eliminate ponding problem is to design and build the floor surface to fall or slope to facilitate water flow. Thus, the expectation of the property owners and architects by not having any water ponding (unless to fall) is not realistic. There must be an acceptance of reasonable tolerance on the level of ponding. What causes water ponding? Large areas of water ponding or small localised ponding known as birdbaths are results of depressions created on the concrete floor surface during concrete strike-off (screeding) and power trowelling. The other reason for water ponding can be resulted by a deflection of a floor slab caused by the weight of the slab itself after the removal of scaffoldings or a possible change of floor profile on an elevated slab after post-tensioning the slab. Idealistically, to avoid water ponding, concrete floor need to be built with a surface profile that is very flat but not level (designed to fall), to cater for water runoff from a higher to lower elevation. However, modern carpark floors are so large that it is impractical to build floors with gradients, as concrete at the centre need to be much thicker to enable water to fall to the lower perimeter scupper drains. Alternatively, the formworks to receive the concrete can be installed to tent-shape so that the concrete can be laid with consistent thickness with falls. However, it is extremely difficult for builders to actually control and do it precisely. As floors are generally designed and built without gradients, any high spots will restrict water to flow freely to any low-lying areas which then trap and hold water, resulting in water ponding. Floor specification and acceptance The QLASSIC standard mentioned that specification for floor surface evenness allowed is 3mm over 1.2-meter starightedge whereas BS8204-2:2003 (refer Table 1) has three classifications of floor flatness for different usages. For example, SR3 specification, which is a 10mm tolerance under 2-meter straightedge is generally recommended for carpark floors. Fig 2 shows how a SR floor is measured using a 2-meter straightedge placed anywhere on the entire floor surface and uses a slip-gauge to measure the gap under the straightedge. This only measures the flatness of the floor but does not measure the level tolerance from the control datum or finished floor level (FFL). As mentioned earlier, flat and level are two inseparable entities, therefore floor level tolerance also needs to be specified for the entire finished floor. For example, +/- 15mm tolerance from the finished floor level is a very stringent specification imposed on levelness control. If a level tolerance of +/- 15mm is also specified from the finished floor level, logically water ponding of up to 30mm may be observed over the entire floor area. Rectification of water ponding Generally, the method of repair is carried put by grinding the high spots that caused water damming and topping up the low areas with suitable cementitious materials. However, if the high areas are grinded down too much, it will cause new ponding areas around the newly filled up areas. As such, rectification works to eliminate water ponding may backfire as most repair areas will looked worse than original and any repairs not carried out professionally may results in delamination. It is near impossible to build a concrete floor with no water ponding. As such, if the depth of water ponding is very little (not more than 10mm deep), it is bearable and acceptable as it can easily be removed. As today's carparks are mostly dry, any slight imperfections on the floor profiles are unnoticeable when dry. Low spots are evident only if waters is present. Understanding of Surface Regularity (SR) Theoretically, a floor specified to SR2 tolerance accepts 5mm water ponding on every localised measurement using a 2-meter straightedge. However, the common misinterpretation of SR2 is assumed to be a +/- 5mm level tolerance from the control datum (level control), which is near to impossible to achieve. As a result, huge spending for repairs are incurred due to such misunderstandings in complying to the SR standard. Another ambiguity of this standard is that it does not indicate how many bumps are allowed if measurements need to be taken. Generally, at least 5% of the floor area should be measured. For example, for a floor area of 500-square meters, twenty-five equally spaced out points need to be selected and measured randomly. Conclusion Without water stagnation on the floor, any low spots may not be noticeable. Any corrective action taken on insignificant ponding areas is unfavourable, as any repair carried out may make the smooth floor appear worse than before. If the repairs is not carried out properly, in addition to patchiness and colour variations, the repaired area may delaminate. The only areas where water ponding is unacceptable are main walk paths, vehicle turning points and areas which may be a safety hazard, causing people to slip and fall or a vehicle to skid. Thank you
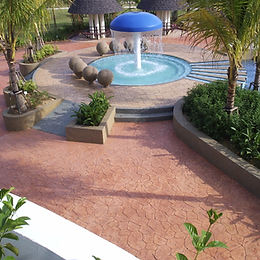
Stamped Concrete Finish in Malaysia – An Introduction
#stampedconcrete #concreteimprint #textureconcrete Stamped concrete popularized in the United States in the 1970s was beginning to make its way to Malaysia in the late 1980s. The systematic method of concrete stamping was introduced by a gentleman named Brad Bowman in the 1950s. After making its foray into Malaysia, this new trend of decorating concrete began slowly to gain momentum in the county. Once dominated by plain concrete finishes, interlocking pavers and floor tiles, today stamped concrete has been trending throughout the county and found favour among the local architects and designers. Stamped concrete exhibits a unique trait in being able to blend a variety of colors and patterns together. This special property is what makes it a popular feature for decorating roads, driveways, walkways, patios and many more places. It also offers a variety of pattern options ranging from wood textures to slate textures. Other benefits of using stamped concrete include among others its affordability, the durability of the product and its requirement of not needing much maintenance. The Early Years In the early years of stamped concrete, the works were largely concentrated in individual luxury houses. In the late 1980s and early 1990s as the trend began to pick up, stamped concrete was being installed in larger volume and scale. Stamped concrete began to feature prominently in many housing developments as it accords a uniqueness to the development. Specifically the stamped concrete works in these developments would generally adopt a single pattern with a two- tone color system to attain a more realistic feel to the design. The blending of colors were typically achieved by combining different products such as colored release powders The Later Years Forty years since stamped concrete was first introduced in Malaysia, it continues to be one of the pillars in both the residential and commercial building sector and is a common product offered among decorative concrete contractors. However, customization and differentiating designs are the new normal in this day and age. Designers and architects are now adding their creative touches to the finishing design by using existing moulds and other decorative products to give it a unique outlook. In order to realize this new feat, contractors are turning to hand tools to create the finer designs such as customized groove and post coloring techniques, for example stains, to blend multiple colors. Challenges The stamped concrete industry do have its challenges. Some of the challenges encountered by decorative concrete contractors often include, among others, issues such as surface delamination, crusting cracks and lack of pristine textures on the final product. Many contractors shared the common problem on surface delamination, though in many ways it is not a reflection of the stamped concrete product itself. The main culprit more often than not is that insufficient time to allow the excessive bleed water to escape before broadcasting the color hardener onto the concrete surface. A little concrete bleeding will be beneficial to facilitate working with the dry shake color hardener but excessive concrete bleeding is one of the causes for delamination when the bleed water and air accumulated under the dense hardened color hardener, unable to escape. Another major cause of delamination is over-layering resulted from broadcasting the color hardener onto hardened concrete, followed by wetting and floating the surface. This issue can be mitigated by adjusting the concrete mix design to reduce concrete bleed water and to improve the workability with sufficient time for finishing. Crusting cracks is another issue seen on some finished works. Whilst the cracks themselves do not generally affect the durability of the concrete slab; their appearance is unsightly on the finished product. These cracks are mainly found near the stamped joints near the edges of the imprinted texture. This occurs as the surface loses water rapidly and has hardened before the rest of the concrete is allowed to set and tends to amplify during hot and windy days. Small cracks are formed around the stamped joints as the stamp mats are pushed into the crusted surface, and the surface tears apart due to stresses. This issue however could minimized by erecting a shade when casting during a hot day and by putting up temporary wind barriers to reduce the wind velocity on the surfaces. Lack of texture on the finished products is a common issue faced in the industry. A major factor that causes this issue is the type of concrete mix used during the stamping process. Concrete mix with large amount of coarse aggregates could interfere with the impression of the stamping process. One way that this could be minimised is to thus ensure the appropriate size and type of aggregates contained in the concrete mix, and in this regard, the experience of the decorative concrete contractor matters. Moving Forward A better understanding of concrete behavior and how the work surroundings could impact upon the final product are essential in bringing up, and capitalizing on, the many great features accorded by decorative stamped concrete products. These factors together with the growing diversity in stamped concrete designs as well as the continuing advancement in concrete technology in Malaysia will be strong sustaining driving forces to propel the decorative stamped concrete industry forward.